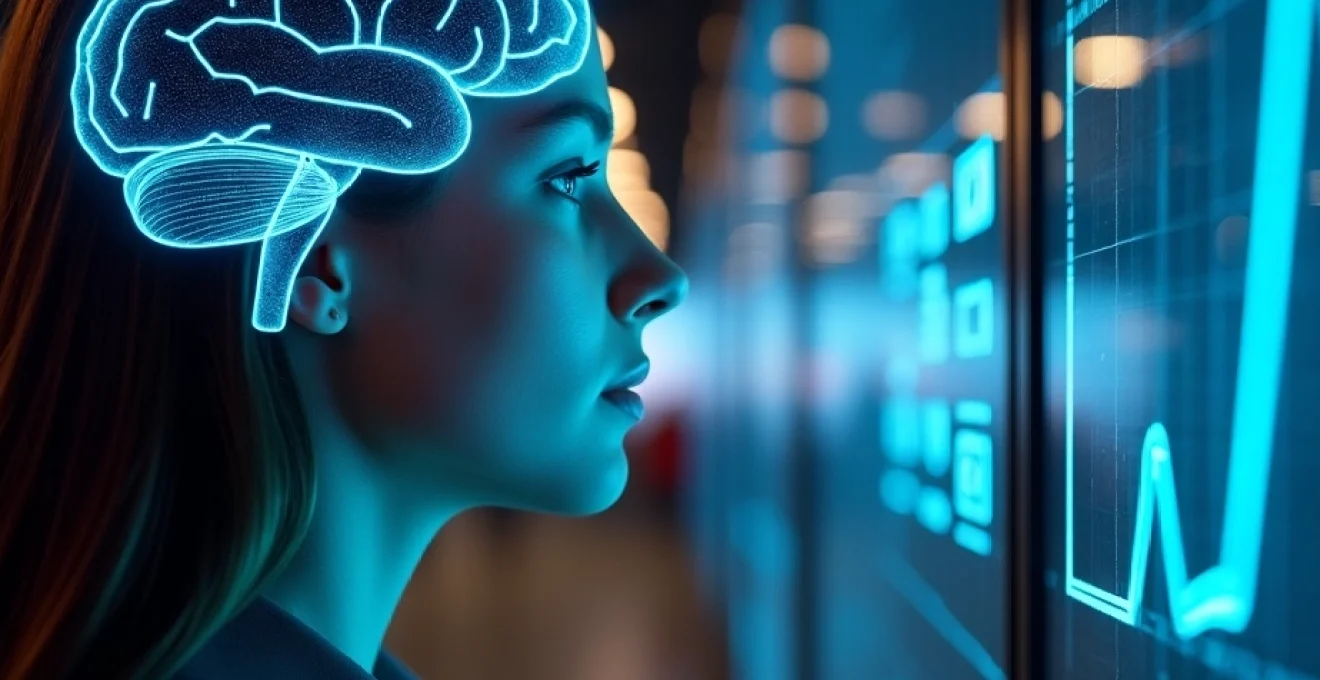
The convergence of neuroscience and engineering has given rise to a groundbreaking field: brain-machine interfaces (BMIs). These innovative systems are poised to revolutionize how humans interact with technology, offering unprecedented control through the power of thought alone. As researchers make rapid strides in decoding neural activity and translating it into machine commands, we stand on the cusp of a new era where the boundaries between mind and machine blur. The implications of this technology stretch far beyond science fiction, promising life-changing applications for individuals with disabilities and opening up exciting possibilities for enhanced human-computer interaction.
Principles of brain-machine interface technology
At its core, BMI technology relies on a sophisticated interplay between the human brain and external devices. By tapping into the brain's electrical signals, these systems aim to create a direct communication channel that bypasses traditional physical interfaces. Understanding the fundamental principles behind BMIs is crucial for grasping their potential and limitations.
Measuring and decoding neural activity
The first step in any BMI system is capturing the brain's neural signals. This process involves sophisticated sensing technologies that can detect the tiny electrical impulses generated by neurons. Electroencephalography (EEG) is a common non-invasive method, using electrodes placed on the scalp to measure overall brain activity. For more precise readings, invasive techniques like electrocorticography (ECoG) or intracortical microelectrode arrays may be employed, offering higher spatial and temporal resolution.
Once these signals are captured, advanced algorithms come into play to decode the neural patterns. Machine learning and artificial intelligence techniques are crucial in this step, helping to identify meaningful patterns amidst the noise of brain activity. These algorithms learn to associate specific neural firing patterns with intended actions or thoughts, creating a 'neural dictionary' of sorts.
Translating brain signals into commands
The decoded neural signals must then be translated into commands that machines can understand and execute. This translation process is where the true magic of BMIs happens. Researchers develop complex models that map neural activity to specific actions or intentions. For example, a particular pattern of brain activity might be interpreted as the intention to move a cursor to the left on a computer screen.
The challenge lies in achieving high accuracy and low latency in this translation process. Ideally, the system should respond to the user's thoughts almost instantaneously, providing a seamless and intuitive experience. This requires not only powerful computing capabilities but also sophisticated algorithms that can quickly process and interpret neural data in real-time.
Feedback loops between brain and machine
A critical aspect of effective BMI systems is the incorporation of feedback loops. These loops allow the user to receive sensory feedback about the actions performed by the machine, closing the circuit between intention and execution. This feedback can come in various forms, such as visual cues on a screen or even direct sensory feedback to the brain itself.
The presence of these feedback loops enables users to refine their control over time, learning to modulate their brain activity more effectively to achieve desired outcomes. It's akin to learning a new skill, where practice and feedback lead to improved performance. In the context of BMIs, this process is often referred to as 'neural plasticity,' highlighting the brain's remarkable ability to adapt and form new neural pathways in response to novel inputs and outputs.
Current research advances in BMI systems
The field of brain-machine interfaces is advancing at a rapid pace, with researchers making significant breakthroughs in various aspects of the technology. Recent studies have demonstrated impressive capabilities, from enabling paralyzed individuals to control robotic limbs to allowing direct brain-to-brain communication between subjects.
One notable advance is in the realm of non-invasive BMIs. Researchers have developed high-resolution EEG systems that can capture more detailed brain activity without the need for surgical implantation. These systems use advanced signal processing techniques to overcome the limitations of traditional EEG, offering improved spatial resolution and reduced signal noise.
In the area of invasive BMIs, miniaturization of electrode arrays has led to devices that can record from thousands of neurons simultaneously. This increased neural coverage allows for more precise decoding of complex motor intentions, bringing us closer to natural, thought-driven control of prosthetic limbs or external devices.
The future of BMIs lies in creating seamless, intuitive interfaces that feel like natural extensions of our cognitive abilities.
Another exciting development is the integration of BMIs with artificial intelligence systems. By leveraging machine learning algorithms, researchers are improving the adaptability and learning capabilities of BMI systems. These AI-enhanced interfaces can learn from the user's brain patterns over time, continuously improving their accuracy and responsiveness.
Challenges in developing mind-controlled devices
Despite the remarkable progress in BMI technology, several significant challenges remain before mind-controlled devices can become a widespread reality. These hurdles span technical, biological, and ethical domains, requiring interdisciplinary approaches to overcome.
Improving signal acquisition and processing
One of the primary technical challenges in BMI development is improving the quality and reliability of neural signal acquisition. Non-invasive methods like EEG suffer from low spatial resolution and are susceptible to external interference. Invasive techniques offer better signal quality but come with risks associated with surgical implantation and long-term biocompatibility issues.
Researchers are exploring novel materials and electrode designs to enhance signal quality while minimizing tissue damage. For instance, flexible electrode arrays that can conform to the brain's surface are being developed to provide stable, long-term recordings. Additionally, advanced signal processing algorithms are being employed to extract meaningful information from noisy neural data, improving the overall performance of BMI systems.
Ensuring system robustness and reliability
For BMIs to be viable for everyday use, they must demonstrate consistent performance across various environments and over extended periods. This requires robust systems that can adapt to changes in neural signals, which can occur due to factors like learning, fatigue, or even minor shifts in electrode positioning.
Developing self-calibrating BMI systems is an active area of research. These systems use machine learning algorithms to continuously adjust their parameters based on the user's brain activity, ensuring optimal performance even as neural patterns evolve over time. Additionally, redundancy in neural decoding algorithms can help maintain reliability in case of partial system failures.
Addressing ethical and safety concerns
The development of BMIs raises important ethical questions that must be carefully considered. Issues of privacy, consent, and potential misuse of neural data are at the forefront of these concerns. How can we ensure that an individual's thoughts remain private when connected to a BMI system? What safeguards are needed to prevent unauthorized access or manipulation of neural interfaces?
Safety is another critical consideration, particularly for invasive BMIs. Long-term studies are needed to assess the potential risks associated with chronic brain implants, including infection, tissue damage, and neurological side effects. Researchers must also address the psychological impacts of using BMIs, such as changes in self-perception or cognitive function.
As we unlock the potential of mind-controlled technology, we must navigate a complex landscape of ethical considerations to ensure responsible development and deployment.
Potential applications of mind-controlled tech
The potential applications of mind-controlled technology are vast and diverse, spanning multiple domains from healthcare to entertainment. As BMI systems continue to improve in accuracy and usability, we can expect to see innovative applications that transform various aspects of our lives.
Assistive technologies for disabled individuals
Perhaps the most impactful application of BMIs is in the realm of assistive technologies for individuals with disabilities. For those with severe motor impairments, mind-controlled devices offer the promise of restored independence and improved quality of life. Some current and potential applications include:
- Thought-controlled prosthetic limbs that provide natural, intuitive movement
- Communication systems for individuals with locked-in syndrome or severe paralysis
- Wheelchair control through brain signals, enabling greater mobility
- Home automation systems that respond to mental commands, allowing control of lights, appliances, and more
These technologies have the potential to dramatically improve the lives of millions of people worldwide, offering new levels of autonomy and interaction with their environment.
Enhanced human-computer interaction paradigms
Beyond assistive applications, BMIs are poised to revolutionize how we interact with computers and digital devices. Imagine a world where you can control your smartphone, navigate virtual reality environments, or interact with smart home devices using only your thoughts. This could lead to more intuitive and efficient interfaces, reducing the cognitive load associated with traditional input methods.
In the workplace, BMIs could enhance productivity by allowing hands-free control of complex systems or enabling rapid information retrieval and decision-making. For instance, a surgeon could access patient data or control robotic surgical tools without breaking sterility, or a stock trader could execute trades with the speed of thought.
Futuristic scenarios in various domains
Looking further into the future, the potential applications of BMIs become even more exciting and transformative. Some speculative but plausible scenarios include:
- Direct brain-to-brain communication, allowing for telepathic-like exchange of thoughts and emotions
- Enhanced learning through direct neural stimulation or memory augmentation
- Immersive entertainment experiences where users can control and interact with virtual worlds through thought alone
- Neural interfaces for AI systems, enabling more intuitive and seamless human-AI collaboration
While these scenarios may seem like science fiction today, the rapid pace of BMI research suggests that they could become reality sooner than we might expect. As with any transformative technology, careful consideration of the ethical and societal implications will be crucial as we navigate this new frontier of human-machine interaction.
Long-term outlook for BMI developments
As we look to the future of brain-machine interface technology, it's clear that we are on the cusp of a new era in human-computer interaction. The long-term outlook for BMI developments is both exciting and challenging, with potential advancements that could fundamentally change how we interact with technology and even how we define human cognition.
One of the most promising areas for long-term BMI development is the integration of artificial intelligence and machine learning. As these technologies continue to advance, we can expect BMIs to become more adaptive and intuitive, learning from each user's unique neural patterns to provide increasingly personalized and efficient interfaces. This symbiosis between human cognition and AI could lead to unprecedented enhancements in problem-solving, creativity, and information processing.
Another area of focus is the development of bi-directional BMIs that not only read neural signals but also provide sensory feedback directly to the brain. This could enable more natural and immersive prosthetic control, allowing users to feel texture, temperature, and pressure through their artificial limbs. In the realm of augmented reality, such bi-directional interfaces could create deeply immersive experiences that seamlessly blend the digital and physical worlds.
Researchers are also exploring the potential of BMIs to enhance cognitive functions such as memory and attention. While still in early stages, studies have shown promising results in using neural stimulation to improve memory formation and recall. As our understanding of the brain's cognitive processes deepens, we may see BMIs that can boost learning, creativity, and even emotional regulation.
The miniaturization and improvement of BMI hardware is another crucial area for long-term development. Future BMIs may utilize nanotechnology to create minimally invasive or even injectable neural interfaces, dramatically reducing the risks associated with current surgical implantation methods. These advanced interfaces could provide higher-resolution neural recordings and more precise stimulation capabilities.
As BMI technology matures, we can expect to see its integration into various aspects of daily life. From enhancing workplace productivity to revolutionizing entertainment and social interaction, the potential applications are vast. However, this widespread adoption will also bring new challenges in terms of privacy, security, and ethical considerations.
The regulatory landscape for BMIs will need to evolve to keep pace with technological advancements. Establishing clear guidelines for the development, testing, and deployment of BMI technologies will be crucial to ensure safety and protect user rights. International cooperation will be necessary to create standardized protocols and ethical frameworks for this rapidly advancing field.